Singular evanescent wave resonances in moving media
Resonators fold the path of light by reflections leading to a phase balance and thus constructive addition of propagating waves. However, amplitude decrease of these waves due to incomplete reflection or material absorption leads to a finite quality factor of all resonances. Here we report on our discovery that evanescent waves can lead to a perfect phase and amplitude balance causing an ideal Fabry-Perot resonance condition in spite of material absorption and non-ideal reflectivities. This counterintuitive resonance occurs if and only if the metallic Fabry-Perot plates are in relative motion to each other separated by a critical distance. We show that the energy needed to approach the resonance arises from the conversion of the mechanical energy of motion to electromagnetic energy. The phenomenon is similar to lasing where the losses in the cavity resonance are exactly compensated by optical gain media instead of mechanical motion. Nonlinearities and non-localities in material response will inevitably curtail any singularities however we show the giant enhancement in non-equilibrium phenomena due to such resonances in moving media.
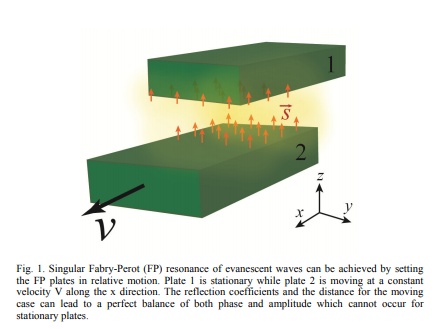
Guo, Yu, and Zubin Jacob. "Singular evanescent wave resonances in moving media." Optics express 22, no. 21 (2014): 26193-26202.
PT-symmetric spectral singularity and negative-frequency resonance
Vacuum consists of a bath of balanced and symmetric positive- and negative-frequency fluctuations. Media in relative motion or accelerated observers can break this symmetry and preferentially amplify negative-frequency modes as in quantum Cherenkov radiation and Unruh radiation. Here, we show the existence of a universal negative-frequency-momentum mirror symmetry in the relativistic Lorentzian transformation for electromagnetic waves. We show the connection of our discovered symmetry to parity-time (PT) symmetry in moving media and the resulting spectral singularity in vacuum fluctuation-related effects. We prove that this spectral singularity can occur in the case of two metallic plates in relative motion interacting through positive- and negative-frequency plasmonic fluctuations (negative-frequency resonance). Our work paves the way for understanding the role of PT-symmetric spectral singularities in amplifying fluctuations and motivates the search for PT-symmetry in novel photonic systems.
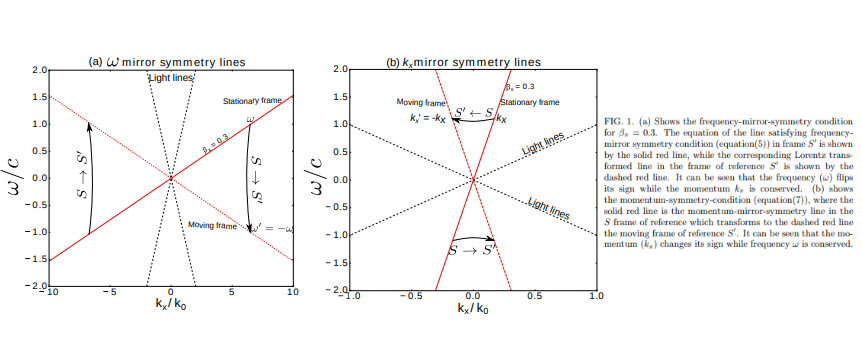
Pendharker, Sarang, Yu Guo, Farhad Khosravi, and Zubin Jacob. "PT-symmetric spectral singularity and negative-frequency resonance." Physical Review A 95, no. 3 (2017): 033817.
Giant non-equilibrium vacuum friction: role of singular evanescent wave resonances in moving media
We recently reported on the existence of a singular resonance in moving media which arises due to perfect amplitude and phase balance of evanescent waves. We show here that the nonequilibrium vacuum friction (lateral Casimir–Lifshitz force) between moving plates separated by a finite gap is fundamentally dominated by this resonance. Our result is robust to losses and dispersion as well as polarization mixing which occurs in the relativistic limit.

Guo, Yu, and Zubin Jacob. "Giant non-equilibrium vacuum friction: role of singular evanescent wave resonances in moving media." Journal of Optics 16, no. 11 (2014): 114023.